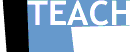 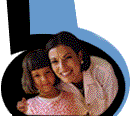

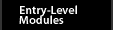

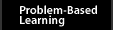
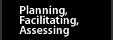
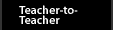

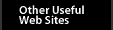 
Resources
for this module
U.S.
Geological Survey
Cascades Volcano Observatory
5400 MacArthur Blvd.
Vancouver, WA 98661
Phone: (206) 696-7693
Fax: 206-696-7866
U.S. Geological
Survey
Earth Science Information Center (ESIC)
678 U.S. Courthouse
W. 920 Riverside Ave., Spokane, WA 99201
Phone: (509) 353-2524
Fax: (509) 456-6115
Washington
State Department of Natural Resources
Division of Geology and Earth Resources Library
1111 Washington St. SE, Room 173,
PO Box 47007, Olympia, WA 98504-7007
ph: (206) 902-1450
fax: (206) 902-1785
email: cjmanson@ u.washington.edu
References
for Volcanic Hazards
General
Volcanic Hazards
Blong, R. J., 1984, Volcanic hazards--A
sourcebook on the effects of eruptions: Academic Press, 424 p.
Crandell, D.
R.; Booth, B.; Kusumadinata, K.; Shimozuru ,D.; Walker, G. P. L.;
Westercamp, D., 1984, Source-book for volcanic-hazards zonation:
UNESCO Natural Hazards 4, 97 p.
Crandell, D.
R.; Mullineaux, D. R., 1975, Technique and rationale of volcanic-hazards
appraisals in the Cascade Range, northwestern United States: Environmental
Geology, v. 1, no. 1, p. 23-32.
Hickson, C.
J., 1992, Volcanic hazards and volcanism in the Canadian Cordillera.
In Bobrowsky, Peter, editor, Geologic hazards in British Columbia--Proceedings
of the Geologic Hazards '91 Workshop: British Columbia Geological
Survey Branch Open File Report 1992-15, p. 35-56.
Swanson, D.
A.; Casadevall, T. J.; Dzurisin, Daniel; Holcomb, R. T.; Newhall,
C. G.; Malone, S. D.; Weaver, C. S., 1985, Forecasts and predictions
of eruptive activity at Mount St. Helens, USA--1975 1984: Journal
of Geodynamics, v. 3, no. 3/4, p. 397-423.
Tilling, R.
I., editor, 1989, Volcanic hazards: American Geophysical Union;
International Geological Congress, 28th, Short Course in Geology,
v. 1, 123 p. *Particularly
informative or the latest resource for this topic or volcano.
Cascade Range
Bacon, C. R., 1983, Eruptive
history of Mount Mazama and Crater Lake Caldera, Cascade Range,
U.S.A.: Journal of Volcanology and Geothermal Research, v. 18, no.
1-4, p. 57-115.
Crandell,
D. R., 1989, Gigantic debris avalanche of Pleistocene age from ancestral
Mount Shasta volcano, California, and debris-avalanche hazard zonation:
U.S. Geological Survey Bulletin 1861, 32 p.
Crandell,
D. R.; Hoblitt, R. P., 1986, Lateral blasts at Mount St. Helens
and hazard zonation: Bulletin of Volcanology, v. 48, no. 1, p. 27-37.
Crandell,
D. R.; Waldron, H. H., 1973, Volcanic hazards in the Cascade Range.
In Tank, R. W., editor, Focus on environmental geology: Oxford University
Press, p. 19-28.
Crandell,
D. R.; Mullineaux, D. R.; Miller, C. D.,1979, Volcanic-hazards studies
in the Cascade Range of the western United States. In Sheets, P.
D.; Grayson, D. K., editors, Volcanic activity and human ecology:
Academic Press, p. 195-219.
Driedger,
C. L.; Kennard, P. M., 1986, Ice volumes on Cascade volcanoes--Mount
Rainier, Mount Hood, Three Sisters, and Mount Shasta: U.S. Geological
Survey Professional Paper 1365, 28 p., 6 pl.
Harris,
S. L., 1986, The other Cascade volcanoes--Historic eruptions at
Mount St. Helens' sister peaks. In Keller, S. A. C., editor, Mount
St. Helens--Five years later: Eastern Washington University Press,
p. 20-33.
Harris,
S. L., 1988, Fire mountains of the West--The Cascade and Mono Lake
volcanoes: Mountain Press Publishing Company, 379 p.
Hoblitt,
R. P.; Miller, C. D.; Scott, W. E., 1987, Volcanic hazards with
regard to siting nuclear-power plants in the Pacific Northwest:
U.S. Geological Survey Open-File Report 87-297, 196 p., 5 pl. *Particularly
informative or the latest resource for this topic or volcano.
Norris,
R. D., 1991, The Cascade Volcanoes--Monitoring history and current
land management: U.S. Geological Survey Open-File Report 91-31,
89 p.
Richardson,
Donald, 1968, Glacier outburst floods in the Pacific Northwest:
U.S. Geological Survey Professional Paper 600-D, p. D79-D86.
Weaver,
C. S.; Norris, R. D.; Jonientz-Trisler, Chris, 1990, Results of
seismological monitoring in the Cascade Range, 1962-1989 Earthquakes,
eruptions, avalanches, and other curiosities: Geoscience Canada,
v. 17, no. 3, p. 158-162.
Glacier Peak
Beget, J. E., 1981, Postglacial
eruption history and volcanic hazards at Glacier Peak, Washington:
University of Washington Doctor of Philosophy thesis, 192 p.
Beget,
J. E., 1982, Postglacial volcanic deposits at Glacier Peak, Washington,
and potential hazards from future eruptions: U.S. Geological Survey
Open-Pile Report 82-830, 77 p. *Particularly informative or the
latest resource for this topic or volcano.
Beget,
J. E., 1982, Recent volcanic activity at Glacier Peak: Science,
v. 215, no. 4538, p. 1389-1390.
Beget,
J. E., 1983, Glacier Peak, Washington--A potentially hazardous Cascade
volcano: Environmental Geology, v. 5, no. 2, p. 8392.
Beget,
J. E., 1984, Tephrochronology of late Wisconsin deglaciation and
Holocene glacier fluctuations near Glacier Peak, north Cascade Range,
Washington: Quaternary Research, v. 21, no. 3, p. 304-316.
Mount Adams
Hildreth, Wes; Fierstein, Judy, 1985, Mount
Adams--Eruptive history of an andesite-dacite stratovolcano at the
focus of a fundamentally basaltic volcanic field. In Guffanti, Marianne;
Muffler, L. J. P., editors, Proceedings of the workshop on geothermal
resources of the Cascade Range: U.S. Geological Survey Open-File
Report 85-521, p. 44-50.
Vallance, J.
W., 1986, Late Quaternary volcanic stratigraphy on the southwestern
flank of Mount Adams volcano, Washington: University of Colorado
Master of Science thesis, 122 p.
Vallance, J.
W., [in press], Postglacial lahars and potential hazards in the
White Salmon River system on the southwest flank of Mount Adams,
Washington: U.S. Geological Survey Bulletin.
Mount Baker
Cary, C. M.; Thompson, J. M.
S.; Pringle, P. T., 1992, Holocene lahar deposits from Mount Baker
volcano in Glacier Creek, North Cascades, Washington [abstract]:
Geological Society of America Abstracts with Programs, v. 24, no.
5, p. 13.
Easterbrook,
D. J., 1975, Mount Baker eruptions: Geology, v. 3, no. 12, p. 679-682.
Frank,
D. G., 1983, Origin, distribution, and rapid removal of hydrothermally
formed clay at Mount Baker, Washington: U.S. Geological Survey Professional
Paper 1022-E, 31 p., 1 pl.
Frank,
D. G.; Meier, M. F.; Swanson, D. A.; and others, 1977, Assessment
of increased thermal activity at Mount Baker, Washington, March
1975-March 1976: U.S. Geological Survey Professional Paper 1022-A,
49 p.
Frank,
D. G.; Post, A. S.; Freidman, J. D., 1975, Recurrent geothermally
induced debris avalanches on Boulder Glacier, Mount Baker, Washington:
U.S. Geological Survey Journal of Research, v. 3, no. 1, p. 77-87.
Hyde,
J. H.; Crandell, D. R., 1978, Postglacial deposits at Mount Baker,
Washington, and potential hazards from future eruptions: U.S. Geological
Survey Professional Paper 1022-C, 17 p., 1 pl. *Particularly informative
or the latest resource for this topic or volcano.
Mount Hood
Cameron, K. A.; Pringle, P.
T., 1986, Post-glacial lahars of the Sandy River basin, Mount Hood,
Oregon: Northwest Science, v. 60, no. 4, p. 225-237.
Cameron,
K. A.; Pringle, P. T., 1987, A detailed chronology of the most recent
major eruptive history of Mount Hood, Oregon: Geological Society
of America Bulletin, v. 99, no. 6, p. 845-851.
Cameron,
K. A.; Pringle, P. T., 1991, Prehistoric buried forests of Mount
Hood: Oregon Geology, v. 53, no. 2, p. 34-43.
Crandell,
D. R., 1980, Recent eruptive history of Mount Hood, Oregon, and
potential hazards from future eruptions: U.S. Geological Survey
Bulletin 1492, 81 p., 1 pl. *Particularly informative or the latest
resource for this topic or volcano.
|
|

Volcanic
Hazards: A Growth Management Perspective
Patrick
T. Pringle
Washington State Department of Natural Resources
Division of Geology and Earth Resources
PO Box 47007, Olympia, WA 98504-7007
Washington is home to
five major composite volcanoes or stratovolcanoes: Mount Baker,
Glacier Peak, Mount Rainier, Mount St. Helens, and Mount Adams.
These volcanoes and Mount Hood to the south in Oregon are part of
the Cascade Range, a volcanic arc that stretches from southwestern
British Columbia to northern California.
Although there are thousands
of small basaltic or basaltic-andesitic volcanoes in the Cascade
Range, the 13 major composite volcanic centers in the U.S., all
part of the range, have been the focus of most hazard concerns.
During the past 12,000 years, these volcanoes have produced more
than 200 eruptions that have generated tephra (ejected material),
lava flows, lahars (volcanic debris flows) and debris avalanches
(Miller, 1990). It is important to note that other enormous debris
avalanches and lahars may have been caused by intrusions of magma
(not eruptions) or steam explosions at the volcanoes or by local
or regional earthquakes because these flowage events do not correlate
with known tephra layers.
All Washington volcanoes
except Mount Adams have erupted within the last 250 years (Table
1). Mount Hood erupted in the late 1700s and into the mid-1800s
(Crandell, 1980; Cameron and Pringle, 1987). However, the volcanoes
do not erupt at regular intervals, thus making it difficult to forecast
when a given volcano might come to life again. Although, worldwide,
the risks from volcanoes are significantly lower than risks from
earthquakes and landslides. The relatively long recurrence interval
for volcanic hazards (decades to several centuries) combined with
their great potential for destruction make them particularly insidious.
This article will focus
mainly on the volcanic hazards associated with the five Washington
volcanoes and Mount Hood, although other volcanic areas, such as
the Indian Heaven volcanic field southwest of Mount Adams, have
erupted during the Holocene (10,000 years ago to present). Also,
this article briefly reviews the terminology used to communicate
volcanic hazards information and provides an overview of volcanic
processes and hazards in Washington State, with particular emphasis
on how they relate to the Washington Growth Management Act (GMA).
Additional information resources are listed at the end of the article.
Volcanic
Hazards and the Planning Process
The GMA mandates
comprehensive planning in some jurisdictions. This mandated planning
effort includes delineation of geologically hazardous areas (including
volcanic hazard areas) as the initial planning step and subsequent
formulation of regulations governing development in hazardous areas.
To this end, in December of 1990, the Department of Community Development
(now the Department of Community, Trade and Economic Development)
issued minimum guidelines for classifying volcanic hazards with
respect to the GMA:
Volcanic hazard areas
shall include areas subject to pyroclastic flows, lava flows, debris
avalanche, inundation by debris flows, mudflows or related flooding
resulting from volcanic activity.
Certain volcanic hazards,
such as fallout of tephra and tsunamis, although important, were
not included in the guidelines. Tephra fallout hazards, for example,
are extremely widespread in volcanic terrains, and the proximal
hazard zones shown in Figure
1 already include the areas that have the highest probability
of destructive tephra fallout. Hoblitt and others (1987) discuss
tephra hazards with respect to the siting of nuclear power plants,
and Casadevall (1992, 1993) addresses the problem of volcanic ash
and aviation safety.
As will be noted below,
evidence for the hazardous volcanic processes mentioned in the GMA
guidelines has been observed in the geologic records of various
Cascade volcanoes (Crandell and Waldron, 1973; Miller, 1990). Before
the cataclysmic eruption of Mount St. Helens in 1980, roughly 30
person-years had been devoted to analysis of volcanic hazards in
the Cascade Range. While much has been learned about the history
of the Cascade volcanoes because of these early studies, more geologic
investigations are necessary in order to bring some of the older
hazard assessments up to date with our current understanding of
volcanic processes. Furthermore, some inconsistencies exist in the
understanding of hazards from individual volcanoes because some
(such as Mount St. Helens) have been studied in considerably greater
detail than others (for example, Glacier Peak and Mount Baker).
Volcanic hazards are
destructive volcanic processes that have a definite probability
of occurring. In order to best understand the importance of considering
volcanic processes and hazards in the planning process, it is helpful
to briefly review the concept of risk and the magnitude of the loss.
Risk can be expressed as a function of the hazard, value that could
be lost (lives, property, stock, etc.) and vulnerability (generally
speaking, preparedness). Using this definition, it is all too clear
that the number of lives and amount of property at risk to volcanic
hazards in Washington is rapidly growing as development spreads
into hazardous areas, such as valleys that drain active volcanoes.
While most of these communities had at least twice the population
in 1990 that they had in 1950, at least one, Kent, has had a ten-fold
increase!
Volcanic
Processes and Hazardous Volcanic Events
The following
discussion focuses on volcanic processes and hazards, particularly
those mentioned in the GMA guidelines. For a more complete discussion
of this topic, Chapter 2 in Tilling (1989) is an excellent reference.
Generally, composite
volcanoes are associated with subduction zones. Consequently, they
are found around the Pacific Rim ("Ring of Fire") and
in the Mediterranean-Himalayan Belt. They are mainly characterized
by the following processes, features, or aspects.
Lava Domes and Flows
Lavas of higher viscosity
tend to pile up and form domes because they cannot flow as readily
as those having lower viscosity (such as Hawaiian basaltic lavas).
The new Lava Dome at Mount St. Helens was constructed during 17
episodes of activity from 1980 to 1986 that included both intrusion
of new magma into the dome and extrusion of lobes of lava onto the
dome's surface and flanks. Crater Rock at Mount Hood is a remnant
of a dome that was constructed by eruptions during the last 1,800
years. Hazards from lava domes include explosions and collapses.
Dome collapse can produce pyroclastic flows and surges, lahars,
and floods.
The main hazard from
lava flows is damage or total destruction by burying, crushing,
or burning everything in their paths. (However, more viscous lava
flows do not typically flow far from the volcano.) Lava flows can
melt snow and ice, but they generally do not produce major floods
and lahars because they do not mix turbulently with snow and ice.
They can, however, melt large quantities of ice, which can be released
as "jokulhlaups" or glacial outburst floods.
Pyroclastic Density
Currents Pyroclastic
density current is a general name for various types of flows of
hot gas and rock down the slopes of a volcano. These geologic processes,
which include pyroclastic flows, pyroclastic surges, and lateral
blasts, generally only affect areas relatively close to the volcano.
Pyroclastic flows are
masses of hot (300 deg. -800+deg.C), dry pyroclastic debris and
gases that move rapidly along the ground surface at velocities ranging
from ten to several hundred meters per second. Typical pyroclastic
flows have two components: (1) a ground-hugging, dense, basal portion
(the flow); and (2) a preceding or overriding turbulent ash-cloud
surge that has separated from the flow.
Direct hazards of pyroclastic
flows are asphyxiation, burial, incineration, and impact. Pyroclastic
flows also can generate lahars and floods by quickly melting snow
and ice, can dam tributary valleys, and can start fires. Pyroclastic
flows are strongly controlled by topography and are likely to be
restricted to valley floors. Although most pyroclastic flows would
be limited to within about 15 miles (25 km) of a volcano, Heller
and Dethier (1981) noted Holocene pyroclastic flow deposits about
18 miles (30 km) from Mount Baker in the Baker Valley.
Unlike pyroclastic flows,
hot pyroclastic surges, because they are less concentrated and less
dense than pyroclastic flows, are not necessarily confined to valleys
and can affect more extensive areas many tens of kilometers from
the volcano. Hot pyroclastic surges can generate secondary pyroclastic
flows. Surges are responsible for many catastrophes, including 30,000
deaths at Mount Pelee (1902) and 2,000 at El Chichon (1982). Cold,
or base, surges typically result from explosive interactions of
magma with water, such as that at Kilauea, Hawaii, in May of 1924
and, on a smaller scale, the post-1980 phreatic (steam) explosions
on the Pumice Plain and in the crater at Mount St. Helens.
Another possible hazard
from Cascade volcanoes is the directed blast. Directed blasts are
very powerful, laterally directed explosions such as that at Mount
St. Helens in 1980 and at Bezymianny, Kamchatka, in 1956. Blasts
can affect large areas (230 mi2 or 600 km2 at Mount St. Helens).
Lahars, Lahar-Runout
Flows, And Floods Lahars
are volcanic debris flows ("mudflows") or rapidly flowing
mixtures of rock debris mobilized by water that originate on the
slopes of a volcano (Figs.
2 and 3). Most are restricted to stream valleys. Although there
are many causes of lahars, two major flow types have been noted,
granular and muddy.
Granular Lahars
have a relatively low clay content (<4%). These flows typically
begin as a flood surge that incorporates sediment and becomes a
debris flow as it travels. The debris flow then rapidly transforms
downstream to more diluted flow types (lahar-runout flows, floods).
The many causes of these clay-poor lahars include:
Interaction of a pyroclastic
density current with snow and ice
Meteorologically (rainstorm
or rain-on-snow events) induced erosion of tephra (or other fragmental
debris) from the slopes of a volcano
Failure of a landslide-dammed
lake
Glacial outburst flood
(jokulhlaup)
Muddy Lahars are
relatively clayrich (>4%) and typically originate as "sector
collapses" of a significant portion of a volcano. They can
be triggered by earthquakes, steam explosions, intrusions, or by
other destabilization (not necessarily by eruptions). Muddy lahars
can have enormous volumes and flow great distances. The Electron
and Osceola Mudflows at Mount Rainier and the Middle Fork Nooksack
flow at Mount Baker are local examples. The volume of the Electron
Mudflow has been estimated at more than 300 million yd3 (0.25 km3)
and the volume of the Osceola Mudflow at more than 0.7 mi3 (3 km3)
(Scott and others, 1992).
Inundation height, runout
length, velocity, and duration of flood wave for lahars can vary
widely. The elapsed time between events, amount of available sediment
for bulking, and other factors can change the scale of the hazard
from lahars. In general, relative risk decreases with distance down
valley and with height above the valley floor.
Debris Avalanches
are flowing mixtures of rock and soil, with or without water, that
move away from a volcano at high speed. Some of these events are
gigantic (>1018 m3). Since the 1980 eruption of Mount St. Helens,
hummocky debris avalanche deposits have been recognized at several
hundred volcanoes around the world. Some debris avalanches at volcanoes
have been caused by earthquakes (Ontake Volcano, Japan, 1984). Debris
avalanches are an end member of a continuum of mass-wasting processes
at composite volcanoes--large lahars at some highly hydrothermally
altered volcanoes, such as Mount Rainier, have undoubtedly transformed
directly from debris avalanches.
Geologists now realize
that this type of gigantic avalanche and those that transform into
lahars occur far more frequently than previously recognized. The
nature of this hazard and its relation to hydrothermal alteration
and destabilization of a volcanic cone also indicate that sector
collapse events (very large debris avalanches) can affect any drainage
that heads on a volcano. The frequency of enormous megathrust earthquakes
in the Cascadia subduction zone (13 in the past 7,600 years), as
well as our increasing recognition of shallow-crustal fault zones
in the Puget-Willamette Lowland and along the Cascades, amplify
the need to consider this major slope-stability hazard at composite
volcanoes.
The debris avalanche
from Ontake Volcano (mentioned above) exemplifies this type of hazard.
On September 14, 1984, a 6.8 magnitude earthquake triggered a debris
avalanche that traveled about 13 km downvalley, killing 29 people.
Numerous larger slope failures in the geologic record could be attributed
to tectonic sesmicity. These and other uncommon events, such as
the 1980 blast at Mount St. Helens, are now included in hazard assessments
at composite volcanoes.
Secondary
Effects of Eruptions
The secondary
effects of the 1980 Mount St. Helens eruption serve as a reminder
that hazards are present long after the initial eruptive activity
has ceased. At Mount St. Helens, dramatic post-eruption erosion
and sedimentation and the ongoing potential of floods from lakes
impounded by the 1980 debris avalanche have presented costly problems.
Nearly $1 billion was spent during the first 10 years after the
eruption to mitigate the downstream flood hazards.
During the first 3 years
after 1980, an estimated 8 million tons of tephra were washed off
hillslopes into the Toutle River system. While hillslope erosion
eased somewhat after 1983, erosion of the debris avalanche and the
subsequent widening and incision of this drainage system by the
development of a stream network resulted in a huge sediment discharge
to downstream areas. The post-eruption Toutle River became one of
the most sediment-laden rivers in the world. Downstream water quality
and aquatic habitat severely deteriorated, and increased downstream
flooding due to sediment-filled river channels jeopardized homes
and roads built near the river.
Numerous natural dams
were created by the debris avalanche in the North Fork Toutle River
drainage. On at least five occasions from 1980 to 1982, the collapse
of a dam released a small lake or pond and caused minor floods.
However, public concern focused on Spirit, Coldwater, and Castle
Lakes, the three largest lakes impounded by the debris avalanche.
Studies by geologists in the 1980s indicated that enormous floods
had resulted from the breakouts of lakes in similar settings at
the volcano in pre-historic times. These types of secondary hazards
could affect any volcano after an eruption or sector collapse.
Volcanic-Hazard
Assessments and Hazard-Zonation Maps
Volcanic-hazard
assessments for Cascade Range volcanoes provide forecasts of the
type and nature of eruptions and volcanic hazards that we can expect
in the future and the areas that would be affected (Crandell and
others, 1975; Hoblitt and others, 1987). Some of these assessments
apply to a wide variety of hazards at an individual volcano (Crandell
and Mullineaux, 1978), while others apply to a more specific type
of hazard, such as debris flows, and may provide detailed information
about their flow processes, including travel times and volumes (Scott
and others, 1992). Volcanic hazards assessments are possible because
geologists have reconstructed the nature of eruptive activity or
hazard by using historical records and the stratigraphic history
of each of these Cascade volcanoes over the past 10,000 years. These
hazards studies make the assumption that future volcanic activity
at each volcano will be similar in style, scale, and frequency to
its past activity.
Volcanic-hazard zonation
maps (Fig. 4) designate
hazardous areas around a volcano. In the past, these maps have shown
relative hazard (for example, low, medium, and high), although some
recent reports and maps have expressed hazards in probabilistic
terms (Hoblitt and others, 1987; Scott and others, 1992). Table
2 shows an example of probabilistic hazards for lahars at Mount
Rainier. A series of inundation maps based on this investigation
should be available soon (Scott and others, in press).
Hoblitt and others (1987)
found that the most damaging volcanic events occur within 30 miles
(50 km) of a volcano. Therefore, their hazard zonation map shows
a proximal-hazard zone within this 50-km radius of a given volcano
(Fig.4). Because significant
lahars have inundated valley bottoms at distances exceeding 50 miles
(80 km) from Cascade Range volcanoes, Hoblitt and others (1987)
also established distal-lahar and flood-hazard zones for the valley
bottoms that extend along major drainages beyond the 50-km radius
of a volcano. These zones include modern river channels, flood plains,
and low terraces.
Drainages that head on
a volcano are most vulnerable to proximal hazards. Other areas are
less likely to be affected by all but low-probability, high-magnitude
events.
Preparedness
and Mitigation: Public Awareness of Volcanic Hazards
The 1980 events
at Mount St. Helens have changed not only the way volcanic hazards
are studied, but also public awareness of those hazards. The three
most important aspects of volcanic hazards mitigation are: (1) communication
of volcano-monitoring and volcanic-hazards information by geoscientists
to the public, the media, and responsible agencies; (2) emergency
preparedness by responsible agencies and officials; and (3) community
and regional planning and land use designations that minimize the
amount of risk. These three aspects are interrelated. Successful
mitigation depends on the timely communication of understandable
scientific information about the current state of the volcano, as
well as the nature, extent, implications, and likelihood of the
variety of volcanic processes possible at that volcano.
Communication of scientific
information about the status of a volcano has improved mainly because
geologists have observed Mount St. Helens so closely. Public demand
for prompt and comprehensible technical information and the experience
of working with an accessible volcano, such as Mount St. Helens,
have helped scientists refine the communication process. As a result,
geologists now use three types of public statements when describing
volcanic activity:
Factual statements
provide information but do not anticipate future events.
Forecasts are
comparatively imprecise statements about the nature of expected
activity (typically based on the past history and potential of
a volcano and on geologic mapping).
Predictions
are relatively precise statements about the time, place, nature,
and size of impending activity (usually based on measurements
at the volcano).
These types of public
statements about Mount St. Helens and other volcanoes from Alaska
to the Philippines have been accepted by the media and the public
because they clarify public expectations and understanding of volcanic
events and hazards.
Discussion
Hoblitt and
others (1987) present an excellent compilation and analysis of volcanic
hazards in Washington. They established proximal- and distal-hazards
zones and summarized the existing literature on Cascade Range volcanoes.
Their map (about 1:2,000,000 scale) is a general guide to areas
that could be affected by hazardous volcanic processes. Other larger
scale maps have been, and continue to be published (Scott and others,
in press) and are essential for hazard zonation by planning agencies.
Although not a direct
volcanic hazard, increased liquifaction susceptibility due to earthquakes
is enhanced by the presence of thick deposits of volcanic sand and
gravel and generally saturated conditions in lowland areas downstream
of volcanoes (Palmer and others, 1991; Pringle and Palmer, 1992).
Some valley-bottom areas are susceptible to multiple hazards. Liquefaction
and related ground failure during strong seismic shaking could disrupt
evacuation routes. Thus, if a large debris avalanche and (or) lahar
were to be triggered by a regional earthquake with attendant liquefaction
and ground failures on the valley bottom and valley walls, the combined
results could be disastrous.
Summary
The Washington
State Growth Management Act mandates that jurisdictions designate
volcanic hazard areas in their comprehensive plans. Volcanic hazards
occur with a frequency and on a scale that require that they be
considered in comprehensive land-use plans where relevant.
Abundant scientific literature
is available on the subject of volcanic processes and hazards. Some
Washington volcanoes, such as Mount St. Helens, have been studied
in great detail, while others, such as Mount Baker and Glacier Peak,
have only had reconnaissance studies. As a result, volcanic-hazards
investigations and hazard-zonation maps for individual volcanoes
may be out of date or at scales that are too small to be useful
to local planning agencies. Revised volcanic-hazards assessments
and improved hazard-zonation maps are necessary for future planning
and preparedness efforts.
|
|





 











Reutrn
to the main Volcanoes module page
References
for Volcanic Hazards
Mount
Rainier
Crandell, D. R., 1963, Paradise
debris flow at Mount Rainier, Washington: U.S. Geological Survey
Professional Paper 475-B, p. B135-B139.
Crandell,
D. R., 1963, Surficial geology and geomorphology of the Lake Tapps
quadrangle, Washington: U.S. Geological Survey Professional Paper
388-A, 84 p., 2 pl.
Crandell,
D. R., 1967, Volcanic hazards at Mount Rainier, Washington: U.S.
Geological Survey Bulletin 1238, 26 p.
Crandell,
D. R., 1969 [1970], Surficial geology of Mount Rainier National
Park, Washington: U.S. Geological Survey Bulletin 1288, 41 p., 1
pl.
Crandell,
D. R.,1971, Postglacial lahars from Mount Rainier volcano, Washington:
U.S. Geological Survey Professional Paper 677, 75p.,3pl.
Crandell,
D. R., 1973, Map showing potential hazards from future eruptions
of Mount Rainier, Washington: U.S. Geological Survey Miscellaneous
Investigations Series I-836, 1 sheet, scale 1:250,000. *Particularly
informative or the latest resource for this topic or volcano.
Crosson,
R. S.; Frank, David, 1975, The Mt. Rainier earthquake of July 18,
1973, and its tectonic significance: Seismological Society of America
Bulletin, v. 65, no. 2, p. 393-401.
Cullen,
J. M., 1978, Impact of a major eruption of Mount Rainier on public
service delivery systems in the Puyallup Valley, Washington: University
of Washington Master of Urban Planning thesis, 195 p.
Driedger,
C. L., 1988, Geology in action--Jokulhlaups on Mount Rainier: U.S.
Geological Survey Open-File Report 88-459, 2 p.
Driedger,
C. L.; Fountain, A. G., 1989, Glacier outburst floods at Mount Rainier,
Washington State, U.S.A. In Wold, Bjorn, editor, Proceedings of
the symposium on snow and glacier research relating to human living
conditions: Annals of Glaciology, v. 13, p. 51-55.
Fiske,
R. S.; Hopson, C. A.; Waters, A. C., 1963, Geology of Mount Rainier
National Park, Washington: U.S. Geological Survey Professional Paper
444, 93 p.
Frank,
D. G., 1985, Hydrothermal processes at Mount Rainier, Washington:
University of Washington Doctor of Philosophy thesis, 195 p.
Moxham,
R. M.; Crandell, D. R.; Marlatt, W. E., 1965, Thermal features at
Mount Rainier, Washington, as revealed by infrared surveys: U.S.
Geological Survey Professional Paper 525-D, p. D93D100.
Mullineaux,
D. R., 1974, Pumice and other pyroclastic deposits in Mount Rainier
National Park, Washington: U.S. Geological Survey Bulletin 1326,
83 p.
Mullineaux,
D. R.; Sigafoos, R. S.; Hendricks, E. L., 1969, A historic eruption
of Mount Rainier, Washington: U.S. Geological Survey Professional
Paper 650-B, p. B15-B18.
National
Research Council, 1994, Mount Rainier--Active Cascade volcano: National
Academy Press, 114 p.
Pringle,
P. T.; Palmer, S. P., 1992, Liquefiable volcanic sands in Puyallup,
Washington correlate with Holocene pyroclastic flow and lahar deposits
in upper reaches of the Puyallup River valley [abstract]: Geologic
Society of America Abstracts with Programs, v. 24, no. 5, p. 76.
Scott,
K. M.; Pringle, P. T.; Vallance, J. W., 1992, Sedimentology, behavior,
and hazards of debris flows at Mount Rainier, Washington: U.S. Geological
Survey Open-File Report 90-385, 106 p., 1 pl. (To be released as
U.S. Geological Survey Professional Paper 1447-C.) *Particularly
informative or the latest resource for this topic or volcano.
Scott,
K. M. Vallance, J. W.; Pringle, P. T., [in press], Debris flows,
debris avalanches, and floods, at, and downstream from Mount Rainier,
Washington: U.S. Geological Survey Open-File Report 92-654.
Swanson,
D. A.; Malone, S. D.; Samora, B. A., 1992, Mount Rainier-- A Decade
Volcano: Eos (American Geophysical Union Transactions), v. 73, no.
16, p. 177, 185-186.
Swanson,
D. A.; Malone, S. D.; Casadevall, T. J., 1993, Mitigating the hazards
of Mount Rainier: Eos (American Geophysical Union Transactions),
v. 74, no. 12, p. 133.
Mount St. Helens
Collins, B. D.; Dunne, Thomas,
1988, Effects of forest land management on erosion and revegetation
after the eruption of Mount St. Helens: Earth Surface Processes
and Landforms, v. 13, no. 3, p. 193-205.
Crandell,
D. R., 1987, Deposits of pre-1980 pyroclastic flows and lahars from
Mount St. Helens volcano, Washington: U.S. Geological Survey Professional
Paper 1444, 91 p., 1 pl.
Crandell,
D. R.; Mullineaux, D. R., 1978, Potential hazards from future eruptions
of Mount St. Helens volcano, Washington: U.S. Geological Survey
Bulletin 1383-C, 26 p.
Foxworthy,
B. L.; Hill, Mary, 1982, Volcanic eruptions of 1980 at Mount St.
Helens--The first 100 days: U.S. Geological Survey Professional
Paper 1249, 125 p.
Hoblitt,
R. P., 1990, Current perspectives on the 18 May 1980 lateral blast
deposit at Mount St. Helens, Washington [abstract]: Geoscience Canada,
v. 17, no. 3, p. 126.
Lehre,
A. K.; Collins, B. D.; Dunne, Thomas, 1983, Post-eruption sediment
budget for the North Fork Toutle River drainage, June 1980-June
1981. In Okuda, S.; Netto, A.; Slaymaker, O., editors, Extreme land
forming events: Zeitschrift fur Geomorphologie, supplement band
46, p. 143-163.
Lipman,
P. W.; Mullineaux, D. R., editors, 1981, The 1980 eruptions of Mount
St. Helens, Washington: U.S. Geological Survey Professional Paper
1250, 844 p.
Manson,
C. J.; Messick, C. H.; Sinnott, G. M., 1987, Mount St. Helens--A
bibliography of geoscience literature, 1882-1986: U.S. Geological
Survey Open-File Report 87-292, 205 p.
Mullineaux,
D. R., 1986, Summary of pre-1980 tephra-fall deposits erupted from
Mount St. Helens, Washington State, USA: Bulletin of Volcanology,
v. 48, no. 1, p. 17-26.
Pallister,
J. S.; Hoblitt, R. P.; Crandell, D. R.; Mullineaux, D. R., 1992,
Mount St. Helens a decade after the 1980 eruptions--Magmatic models,
chemical cycles, and a revised hazards statement: Bulletin of Volcanology,
v. 54, no. 2, p. 126-146.
Pringle,
P. T., 1993, Roadside geology of Mount St. Helens National Volcanic
Monument and vicinity: Washington Division of Geology and Earth
Resources Information Circular 88, 120 p.
Scott,
K. M., 1988, Origins, behavior, and sedimentology of lahars and
lahar-runout flows in the Toutle-Cowlitz River system: U.S. Geological
Survey Professional Paper 1447-A, 74 p.
Scott,
K. M., 1989, Magnitude and frequency of lahars and lahar runout
flows in the Toutle-Cowlitz river system: U.S. Geological Survey
Professional Paper 1447-B, 33 p.
U.S.
Forest Service, 1992, Mount St. Helens contingency plan 1992--Gifford
Pinchot National Forest: U.S. Forest Service, 1 v.
Yamaguchi,
D. K., 1983, New tree-ring dates for recent eruptions of Mount St.
Helens: Quaternary Research, v. 20, no. 2, p. 246-250.
Miscellaneous References
Bolt, B. A.; Horn, W. L.; MacDonald,
G. A.; Scott, R. F., 1977, Geological hazards--Earthquakes, tsunamis,
volcanoes, avalanches: Springer-Verlag, 330 p.
Casadevall,
T. J., 1992, Volcanic hazards and aviation safety: Federal Aviation
Administration Aviation Safety Journal, v. 2, no. 3, p.917.
Casadevall,
T. J., 1993, Volcanic ash and airports--Discussions and recommendations
from the Workshop on Impacts of Volcanic Ash on Airport Facilities:
U.S. Geological Survey Open-FIle Report 93-518, 52 p.
Dunne,
Thomas; Smith, J. D.; Wigmosta, M. S., 1983, Field evidence for
the flow properties of the Toutle valley mudflows: U.S. Bureau of
Reclamation, 2 sheets microfiche [146 p.]; U.S. National Technical
Information Service PB 86-157138.
Ewert,
J. W.; Swanson, D. A., 1992, Monitoring volcanoes--Techniques and
strategies used by the staff of the Cascades Volcano Observatory,
1980-1990: U.S. Geological Survey Bulletin 1966, 223 p.
Eisbacher,
G. H.; Clague, J. J., 1984, Destructive mass movements in high mountains--Hazard
and management: Geological Survey of Canada Paper 84-16, 230 p.
Fairchild,
L. H., 1985, Lahars at Mount St. Helens, Washington: University
of Washington Doctor of Philosophy thesis, 374 p.
Fairchild,
L. H., 1986, Quantitative analysis of lahar hazard. In Keller, S.
A. C., editor, Mount St. Helens--Five years later: Eastern Washington
University Press, p. 61-67.
Higgins,
J. D.; Naik, Bijayananda; Mills, S. V.; Copp, Howard; Roberson,
J. A., 1983, The mechanics of mudflows: Washington Water Research
Center Report 51, 116 p.
Lopez,
D. L.; Williams, S. N., 1993, Catastrophic volcanic collapse-- Relation
to hydrothermal processes: Science, v. 260, no. 5115, p. 1794-1795.
Norris,
R. D., [in press], Seismicity of rockfalls and avalanches at three
Cascade Range volcanoes--Implications for seismic detection of hazardous
mass movements: Seismological Society of America Bulletin.
Nuhfer,
E. B.; Proctor, R. J.; Moser, P. H., 1993, The citizens' guide to
geologic hazards: American Institute of Professional Geologists,
134 p.
Palmer,
S. P.; Pringle, P. T.; Shulene, J. A., 1991, Analysis of liquefiable
soils in Puyallup, Washington: In Proceedings--Fourth International
Conference on Seismic Zonation, Stanford, California, 1991: Earthquake
Engineering Research Institute, v. 2, p. 621-628.
Stine,
C. M., 1990, Annotated bibliography, volcano-deformation monitoring:
U.S. Geological Survey Open-File Report 90-47, 29 p.
Tanaka,
Janet, 1986, The other side of the volcano--Social aspects of volcanic
activity: Volcano News, no. 25/26, p. 1-8.
Wigmosta,
M. S., 1983, Rheology and flow dynamics of the Toutle debris flows
from Mount St. Helens: University of Washington Master of Science
thesis, 184 p.
Wood,
C. A.; Kienle, J., editors, 1990, Volcanoes of North America: Cambridge
University Press, 354 p.
|
|
 |