What
is the focus of this module?
The purpose of this module is to show students how an event
in one sphere, in this case, the atmosphere, can affect and be affected
by the other spheres, in this case the biosphere, lithosphere, hydrosphere,
and anthrosphere. Students should also obtain a basic understanding
of the mutual feedback that occurs between human activity and the
spheres.
What
is the compelling problem that students will face in this module?
Working in teams,
students will review the many issues that surround ozone depletion.
They will evaluate the current status of the Montreal Protocol,
considering the interrelationships of earth's spheres, including
the anthrosphere. Students must investigate how an event in one
sphere may affect a second sphere, which may, in turn, affect a
third sphere. Students should determine if there is a need for revision
of the Montreal Protocol and, if so, in what areas. Students should
be prepared to defend the advice they give.
What
topics/tasks/issues will students encounter/raise as they work through
this module?
The
main issue that students will likely raise is whether their answers
are correct. For most of the activities (Activity 2 is the exception),
there are no right or wrong answers. Rather, students should support
judgments concerning the correct course of action.
The issue of developing
nations and CFC reduction should be addressed. These nations were
given extra time to come into compliance. The pressing issue now
is how much of the cost of compliance should be born by the developed
countries.
There are social and
humanitarian aspects that should be considered. For example, developing
countries may say, "We are not responsible, why should we have
to pay? Besides, we have more important things to worry about--like
feeding our people."
What
is the role of remote sensing in this module?
The first
remote sensing activity is very straightforward. Students observe
a decrease in ozone concentrations in the higher latitudes during
a 15-year period.
Activity 2 is somewhat
complex and probably beyond the capability of the average student.
Most students will want guidance. On the first day of spring, sun
angle largely determines the amount of radiation incident at the
top of the atmosphere. At the equator, the sun is directly overhead.
At 30° latitude, the sun will make an angle of 30° with the vertical
solar zenith angle, so the top of the atmosphere receives about
86.6% of the radiation received at the equator (cos 30° x total
radiation). At 60° latitude, only 50% of the amount received at
the equator is received . Likewise, the path length the radiation
takes increases with latitude. At 30°, it is 15% more than that
at the equator (sec 30° x path length at equator). This is a multiplier
effect as far as attenuation of radiation is concerned. At 60° latitude,
the path length is doubled, effectively doubling the effect of ozone
at 60° when compared with the equator.
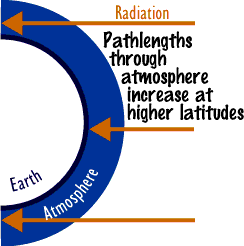
For example, suppose
on the first day of spring, ozone concentrations are 220 Dobson
Units at the equator and 440 Dobson Units at 60° latitude. At 60°,
solar radiation at the top of the atmosphere is half what it is
at the equator. At 60°, path length essentially doubles the effectiveness
of the 440 Dobson Units. Assuming each one percent increase in ozone
reduces uvb radiation by two percent, the ozone at 60° N would reduce
uvb eight times as effectively as the ozone at the equator. The
result is that uvb radiation reaching the ground at 60° is about
1/16 of that at the equator on the first day of spring.
The problem changes at
different times of the year. In the summer, because of longer days
and smaller zenith sun angles, as one approaches the pole, radiation
at the top of the atmosphere is fairly uniform from the tropic of
cancer to about 60° latitude. On the other hand, radiation decreases
very rapidly in the direction of the pole during the winter.
Students may ask the
purpose of activity 3. In activity 3, students should see some relation
between O3, HNO3, and CLONO2 concentrations.
As HNO3 is taken out of the atmosphere in the cold Antarctic
winters and condenses into its liquid form in PSCs, it is no longer
available to react with ClO. ClO is an unstable form of chlorine
important in ozone reduction. When HNO3 undergoes photolysis
in the spring, it is capable of reacting with Cl and ClO, forming
the much more stable form of chlorine, ClONO2. Early
in the spring, much chlorine is available to react with ozone, but
as the HNO3 dissociates, increasing amounts of ClONO2
form. So, after about three weeks the ozone hole begins to close.
Because of atmospheric circulations, there are spatial and temporal
variabilities, so you would not expect to see an exact correlation
at every point plotted.
Preparation
Checklist--have you thought of everything?
|